Introduction | Why is energy storage control important?
Energy storage is key to unlocking the full potential of the existing electricity grid and allowing renewables to generate the bulk of day-to-day power. Until now, in the vast majority of cases, grid operators have only used energy storage solutions as a bolt–on to their existing infrastructure. This helps smooth out some temporary spikes in demand, but still does not provide the bulk of everyday electricity provision. Advanced batteries are expected to play a major role in electricity grid management services for systems with a high share of renewable electricity.
Energy Storage Systems (ESS) aim to provide two basic types of services: power and energy. While most battery systems are optimized to provide one service over another, novel hybrid battery systems, like the hybrid ESS (HESS) being developed in the HYBRIS project, are designed to meet both power and energy service needs. To accomplish this, new methods of controlling battery systems are required, which must be tested to ensure they can be performed safely. Coupling a battery energy storage (BESS) converter controller with a real-time simulation helps speed up development and optimize the design and service offerings in order to comply with certification and standards, such as grid codes.
In microgrids, for example, the battery is critical for managing islanding, synchronizing the grid system, and providing the stored energy necessary to meet grid needs. As such, the ability to island and reconnect to the grid seamlessly is required. This leads to the need to understand fault responses of inverters in power electronics-based resources in grid-connected and islanded modes, which is where hardware-in-the-loop testing comes into its own.
Testing solutions | What is HIL testing?
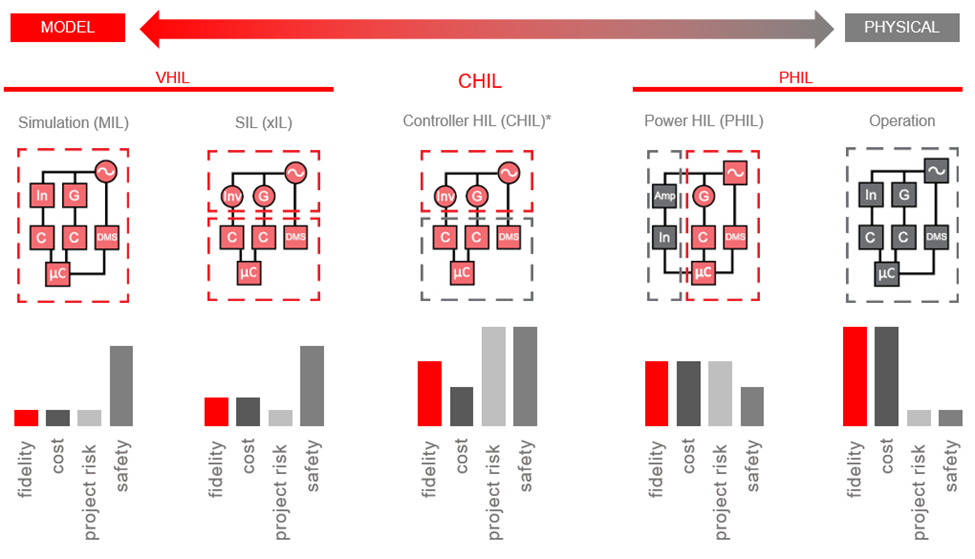
Hardware-in-the-loop, or HIL, testing is when real controller hardware is connected to signals generated by a virtual model of a physical system in real-time. During HIL tests, the hardware device under test, or DUT, “believes” it is operating in the real system, since it receives the same types of signals at the same speed and the same power levels as it would in a real installation. By manipulating the virtual system model during testing, we can validate that the DUT behaves as expected during both normal operational conditions as well as during rare events, such as grid-faults or reverse power flow conditions.
Commonly, HIL testing is divided into two types: Power hardware-in-the-loop (P-HIL) and controller hardware-in-the-loop (C-HIL), corresponding to the right and center of Figure 1. Power hardware-in-the-loop involves testing the real device, such as a battery, connected to power amplifiers that ensure it receives the same power levels it would face under real conditions. This is ideal for final validation of a new system but can be quite expensive and poses safety risks since real power is used.
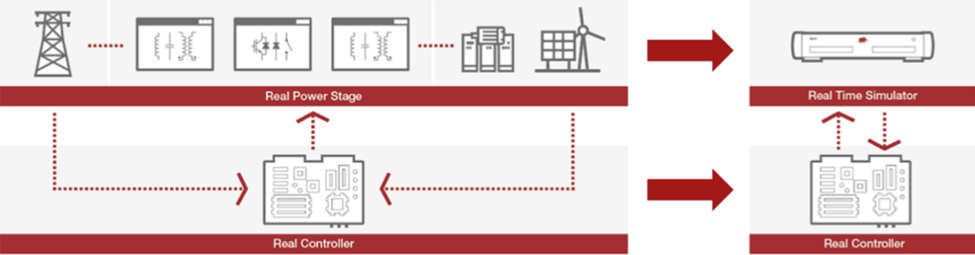
Controller hardware-in-the-loop instead replaces the complete power stage of the DUT with a virtual model, as shown in Figure 2. Real power flows are replaced with digital signals in the power stage model in the simulation, which the controller interprets and then sends control signals to. In this way, control software behavior can be tested in real-time with microsecond or even nanosecond level resolution. This technique is the gold standard for developing, validating, and troubleshooting complex control, protection, and monitoring systems, as it allows you to test real controllers in real conditions without the safety risks posed by high power flows.
HIL solutions | Why HIL?
Electrical systems must always be in balance – a sufficiently severe spike in power demand over less than a second can be enough to catastrophically damage costly equipment. With the HIL Testing concept, these technical and commercial problems can be solved much more efficiently. While simplified models can play a key role in quickly finding potential designs for battery systems, the real-time testing that HIL provides is critical for ensuring that there is not a moment where these systems will fail under the tested conditions. In the HYBRIS project, this is particularly useful for ensuring the converter controller signals do not damage the real battery system, reducing the risk of damaging the prototype battery during development.
HIL can also be useful as a communication tool between equipment manufacturers, product integrators, solution development partners, and final customers. The real-time simulation environment used during HIL tests can help analyze and demonstrate constraints on safe settings for the electronics, without damaging any part of the test bench. HIL technology can therefore provide a high-fidelity simulation of a wide range of failures, verifying that the control software behaves accordingly. This means the right components can be chosen, sized, integrated, and the control software for them tested and verified. In HYBRIS, HIL testing of the battery control system utilizing high-fidelity virtual models of both the battery system and the grid allows us to parameterize and size the HESS battery system to best fit the conditions at a real pilot site.
Finally, HIL testing can satisfy the “digital twin” concept provided the real-time models in the simulation environment are sufficiently high-fidelity that they are essentially indistinguishable from a real system. Creating real-time models with this level of fidelity requires capturing everything from the configuration of the devices used at the site to the communication protocols and communication delays that interact between them. The HYBRIS project seeks to build on this concept by feeding real data from a real site into a high-fidelity HIL model to create a virtual demonstration site – we will cover more on that next month.
Written by Sergio Costa and Eleni Apostolidou, from Typhoon HIL.